Advancements in Sustainable Bioplastics: The Role of Polylactic Acid (PLA) in Environmental Conservation
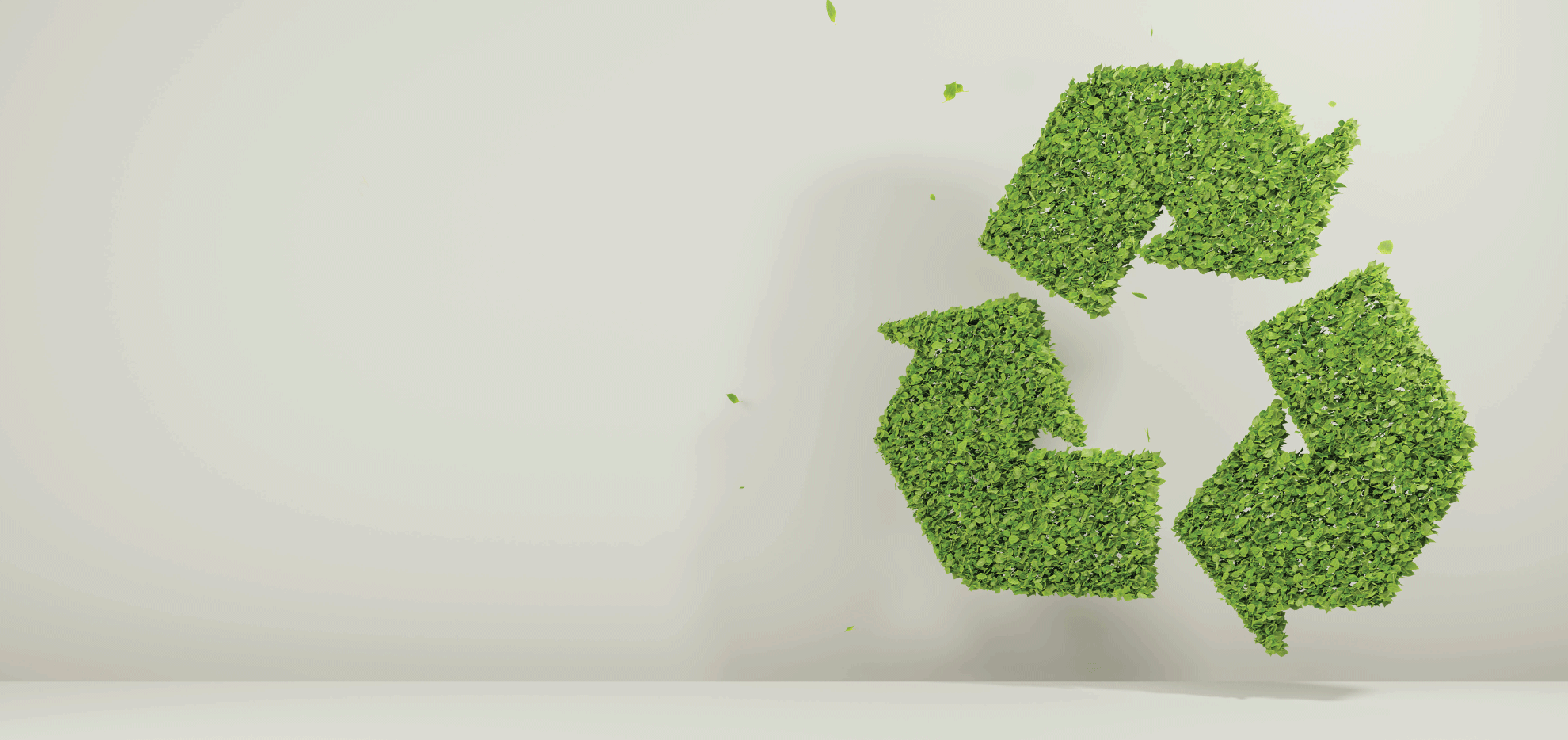
Introduction
Polymers consist of repetitive monomeric units or building blocks interconnected in polymeric chains. These large molecules are typically produced through polymerisation, polycondensation, or polyaddition reactions, traditionally sourced from fossil-based materials. Examples of fossil-based plastics are polyethene (PE), polyethene terephthalate (PET), polystyrene (PS), polypropylene (PP), polyamides (PAs), acrylonitrile–butadiene–styrene (ABS), and others. These polymers are made from petrochemicals and create challenges such as water pollution, chemical pollution, greenhouse gas emissions, contributing to global warming, etc.; there’s a rising interest in competitive biodegradable materials to curb environmental pollution and address waste management challenges. The degradation of plastics in the natural environment releases micro- and nano-plastics. These particles can adversely affect not only aquatic ecosystems but also human health. Consequently, biodegradable plastics, referred to as ‘bio-based polymers’ or ‘bioplastics’, have been suggested as a possible remedy to alleviate the harmful consequences of petrochemical plastics on the environment and human health.
Bioplastics
Bioplastics represent a modern era of plastics, originating from biological systems and production from renewable sources or various microorganisms. These bioplastic materials derive from renewable biological sources such as plants, bacteria, or other organic materials. They can be produced from multiple feedstocks, including starch, corn, sugarcane, lignocellulosic components and vegetable oils. Bioplastics are designed to be biodegradable or compostable, reducing their environmental impact compared to conventional plastics. While they contribute to minimising the reliance on non-renewable resources, it’s essential to consider factors such as production methods, end-of-life disposal, and overall environmental footprint when evaluating the sustainability of bioplastics.
Figure 1 depicts different categories of bioplastics, classified as follows:
- Bio-based or partially bio-based plastics (like bio-PE (PP), biobased PET, PTT)
- Bio-based and biodegradable plastics (like PLA, PHA, and Starch blends)
- Fossil resources and biodegradable plastics (like PBAT, PBS, PCL)
Where: PET – Polyethylene Terephthalate; PPT- Polytrimethylene Terephthalate; PHA – Polyhydroxy Alkanoates; PLA – Polylactic acid; PBS – Polybutylene Succinate; PHB – Poly hydroxybutyrate; PHBV – Poly hydroxybutyrate-co-valerate; PHV – Poly Hydroxy Valerate; Bio-PBS Bio-based polybutylene succinate; Plastics based on starch, cellulose, lignin, and chitosan; PCL – Polycaprolactone
Figure 1: Classification of Bioplastics
Polylactic Acid
Polylactic acid (PLA) is a biodegradable polymer that has received significant attention due to its unique properties and eco-friendly nature. It has a glass transition temperature of 55°C and a melting temperature of around 170°C. Moreover, PLA demonstrates hydrophobic properties relative to other polyesters. Dioxane and chloroform are considered the most suitable solvents for PLA. Regarding permeability coefficients, PLA shows lower CO2, O2, N2, and H2O values than polystyrene (PS) but higher values than PET.
PLA can be produced through fermentation of sugars derived from crops like corn, sugarcane, or tapioca. PLA exhibits various mechanical properties, making it suitable for multiple applications. It can be processed into films, fibres, and moulded parts, making it versatile for packaging, textiles, medical devices, and 3D printing. In addition, It has high transparency and gloss, making it an attractive option for packaging applications where product visibility is essential. Its clarity resembles that of conventional plastics like PET (polyethene terephthalate). While PLA has lower heat resistance than some petroleum-based plastics, advancements in PLA formulations have improved thermal stability. Modified PLA grades can withstand higher temperatures, expanding its range of applications.
Generally, PLA demonstrates better thermal processability than other biopolymers. It lends itself to various processing techniques, such as injection moulding, film extraction, blow moulding, thermoforming, fibre spinning, and film forming. Moreover, PLA typically demands 25-55% less energy for processing than polymers derived from petroleum [1]. PLA has received approval for food contact applications from regulatory agencies such as the U.S. Food and Drug Administration (FDA) and the European Food Safety Authority (EFSA).
PLA properties can be tailored through modifications such as copolymerisation with other monomers or blending with other polymers. These modifications enable the optimisation of PLA for specific applications and performance requirements. However, the production of PLA polymer faces several challenges, such as
- PLA is a relatively hydrophobic material. Hence, it has low cell affinity and can lead to an inflammatory response upon direct exposure to biological fluids.
- PLA exhibits chemical inertness, presenting surface functionalisation and bulk modification challenges.
- Processing challenges include sensitivity to temperature, shear rate, and moisture content during processing.
- Raw material availability, primarily sourced from crops like corn or sugarcane, raises concerns regarding food security.
- High production costs stemming from the complexity and energy intensity of the manufacturing process impact overall production expenses.
- Investing in large-scale PLA production facilities demands substantial capital investment.
Addressing these challenges requires collaboration among stakeholders across the value chain, including raw material suppliers, manufacturers, researchers, policymakers, and consumers. Continued innovation, technological advancements, and supportive policies are essential to overcome barriers and realise PLA’s potential as a sustainable polymer solution.
Process
The process begins with preparing a substrate from a mixture of glucose and demineralised water, which is then sent into a fermentor. Following fermentation, the resulting slurry is directed into a hydro-cyclone, where it undergoes separation into liquid and biomass particles or components. The separated liquor is combined with lime to yield calcium lactate, subsequently undergoing filtration. The calcium lactate is then directed to a lactic acid solubilisation unit, where lactic acid is generated.
Next, the lactic acid undergoes a series of purification steps. It is first sent to a liquid-liquid extraction column and then to a distillation column to enhance its concentration by separating it from other components, such as water. The crude lactic acid, typically around 80-90% purity, is directed into an oligomerisation reactor with a catalyst, followed by a vapour-liquid separator drum to separate lactide. The produced lactide is then directed into a distillation column to obtain pure lactic acid, which is subsequently routed to a polymerisation unit for further processing. In general, there are three methods for the polymerisation of lactic acid: (a) direct condensation polymerisation, azeotropic dehydrative condensation, and polymerisation through lactide formation. Among these methods, direct condensation polymerisation is considered affordable and expensive. However, the challenge of obtaining solvent-free PLA hinders its industrial acceptability. Moreover, efforts to address this challenge by adding coupling agents and adjuvants increase the costs and complexity of the process [2].
Global Status for Bioplastic
Around 97-99% of these plastics originate from fossil fuel feedstock, while the remaining 1-3% are obtained from bio-based plastics.
Globally, around 450 million tonnes of plastic were produced annually in 2020. Approximately 97-99% of these plastics are derived from fossil fuel feedstock, while the remaining 1-3% are produced feedstock [3]. Figure 2 illustrates feedstock biopolymer production, accounting for approximately 4000-4500 kTPA of bio-polymer. China’s total PLA production capacity is approximately 160 kt/year.
Figure 2: Status of production of biopolymer by feedstocks
Major players in the biopolymer industry are actively seeking out countries with abundant agricultural resources and favourable government policies to establish biopolymer manufacturing facilities. Examples of such countries include Thailand, China, France, Brazil, Spain, and the USA. For instance, Dow and UPM Biofuels (UPM BioVerno) have recently announced the commercialisation of bio-based polyethene plastic for packaging applications. LyondellBasell has partnered with Finland-based energy company Neste to produce bio-based propylene and low-density polyethene for food package applications. In collaboration with Global Bioenergies, Clariant has developed isobutene-based polymers used in personal care formulations. Nouryon aims to produce bio-based polymers from itaconic acid for consumer care products, utilising Itaconix’s technology. BiologiQ’s NuPlastiQ has introduced a new potato starch-based material for producing thermoplastic resins, often used to blend with synthetic resins such as polyolefins to enhance their properties. NatureWorks is expanding its worldwide manufacturing presence by constructing a new integrated low-carbon PLA plant in Thailand. With an annual production capacity of 75 KTPA of biopolymer using Ingeo Technology, this facility is anticipated to commence operations by 2024. It will employ cutting-edge biopolymer technology utilising the Lactic Acid route, with raw materials procured from Thai sugarcane farmers, supplying approximately 110 KTPA of sugar.
In India, single-use plastic products crafted from biopolymers are readily accessible. Their utilisation is steadily rising, aligning with growing public awareness and the implementation of effective government policies. Recently, M/S. Praj Industries is establishing a pilot plant for PLA, which is nearing completion and expected to commence operations by 2024.
Takeaways
Bioplastics are designed to be biodegradable or compostable, reducing their environmental impact compared to conventional plastics. They can be produced from various renewable feedstocks, including starch, corn, sugarcane, lignocellulosic components, and vegetable oils. While bioplastics contribute to minimising reliance on non-renewable resources, considerations such as production methods, end-of-life disposal, and overall environmental footprint are essential for evaluating their sustainability. Among bioplastics, Polylactic Acid (PLA) distinguishes itself as a biodegradable polymer derived from corn starch or sugarcane. PLA exhibits a wide range of mechanical properties, making it suitable for diverse applications in packaging, textiles, medical devices, and 3D printing. Despite its eco-friendly nature, PLA production faces challenges such as hydrophobicity, chemical inertness, processing difficulties, raw material availability, high production costs, and capital investments.
From the view of an engineering consultancy firm, the focus is on developing innovative processes for biopolymer production, prioritising efficiency and sustainability. This involves selecting and designing specialised equipment to meet the specific needs of biopolymer manufacturing, aiming to optimise performance and reliability. Additionally, the firm enhances yield, quality, and cost-effectiveness through process optimisation in biopolymer production. Furthermore, they facilitate production scale-up from lab-scale to commercial operations, enabling mass production and market expansion.
References
- Tommaso Casalini, V. L. (2019). A Perspective on Polylactic Acid-Based Polymers Use for Nanoparticles Synthesis and Applications. Front. Bioeng. Biotechnol. https://doi.org/10.3389/fbioe.2019.00259.
- Sreekumar K, B. B. (2021). Perspectives of polylactic acid from structure to applications. Polymers from Renewable Resources. 12(1-2):60-74. doi:10.1177/20412479211008773.
- K. Saraswat, e. a. (2022). Report on Alternative Products and Technologies to Plastics and their Applications. NITI Aayog.