Revolutionising Hydrogen Production: The Promise of Natural Gas Pyrolysis
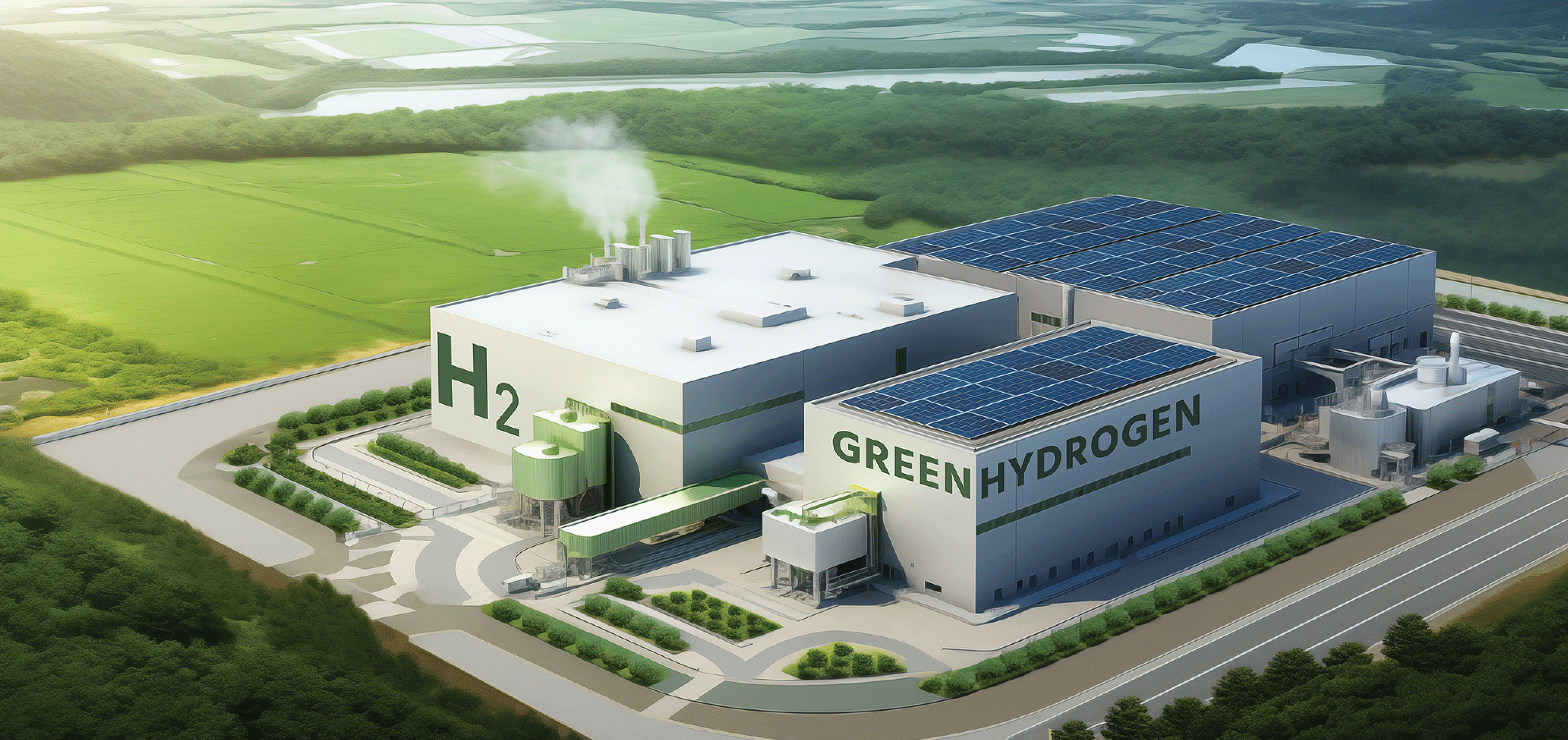
Pyrolysis is a thermochemical process involving the decomposition of organic materials at elevated temperatures without oxygen. In the context of hydrogen production, natural gas pyrolysis refers to the thermal decomposition of methane (the primary component of natural gas) into hydrogen gas and solid carbon. This method offers a promising approach to generating hydrogen due to its simplicity, potential for high-purity hydrogen production, low carbon emissions, and scalability. Natural gas is a significant feedstock for hydrogen production via pyrolysis due to its abundance, accessibility, and relatively low cost compared to other feedstocks. Additionally, the existing natural gas extraction, transportation, and distribution infrastructure makes it a practical choice for large-scale hydrogen production projects. However, it’s important to consider the environmental impact of natural gas utilisation, including carbon emissions associated with methane pyrolysis and the need for carbon capture and utilisation strategies to mitigate these emissions. The primary objective of this article is to examine the viability of hydrogen production through natural gas pyrolysis in comparison to alternative technologies, considering factors such as energy requirements, CO2 emissions, costs, and other relevant metrics.
Fundamentals of Methane Pyrolysis
Methane pyrolysis implies the thermal decomposition of methane (CH4) into its essential elements, mainly hydrogen (H2) and solid carbon (C). It takes place in the absence of oxygen to prevent combustion and ensure the selective production of hydrogen. Methane has the potential to undergo direct conversion into C2 hydrocarbons via methods such as pyrolysis or thermal coupling. This conversion process is endothermic, necessitating high-temperature heat input. The resulting products predominantly include ethene, ethyne, benzene, and hydrogen [1].
Natural gas pyrolysis can be categorised into thermal, thermos-catalytic, and plasma pathways.
- Thermal Pyrolysis: It indicates the decomposition of methane at high temperatures in the absence of oxygen. Methane pyrolysis has a potential method for producing carbon oxide (COx)-free hydrogen through the following endothermic reaction [2].
CH4 (g) → C (s) + 2H2 (g), ΔHo = 74.91 kJ / mol
The non-catalytic thermal pyrolysis of natural gas typically operates at temperatures ranging from 1100°C to 1300°C due to the strong C–H bonds and the symmetry of its molecular structure. This higher temperature needs to break off methane and hydrogen selectivity. This process yields hydrogen gas as the primary product alongside carbon as a by-product, recognised for its cost-effectiveness relative to other pyrolysis techniques. The levelized cost of hydrogen (LCOH) is at 2.8 US$/kg H2, the lowest LCOH among the three methods, though this calculation neglects the by-product’s value. However, if the by-product of solid carbon can be traded at 0.4 US$/kg, the LCOH decreases to 1.6 US$/kg H2. Although it is economically viable, the carbon by-product from thermal pyrolysis tends to be of lower quality than alternatives. [3].
- Thermo-Catalytic Pyrolysis: It involves using a catalyst to facilitate the decomposition of natural gas at lower temperatures (in the range of 800-900 °C) compared to thermal pyrolysis. The catalyst helps lower the activation energy required for the reaction, thereby improving efficiency and selectivity. This approach provides enhanced control over the morphology and quality of the by-product of solid carbon, potentially yielding higher-value outputs such as graphite instead of carbon black. Extensive research has investigated metal and non-metal catalysts (particularly carbon-based materials). Transition metals such as Ni, Fe, and Co are particularly notable in methane pyrolysis due to their heightened activity, optimal operational temperatures, and the prospect of generating valuable carbon nanotubes as a by-product. However, the carbon by-product can be contaminated with the active metal when catalysts like nickel or cobalt are used, posing risks due to their toxicity. Therefore, there is a need for more cost-effective and safer catalysts (that’s iron materials) to make methane pyrolysis feasible for industrial applications.
- Plasma Pyrolysis: Plasma pyrolysis uses ionised plasma to decompose natural gas into hydrogen and solid carbon. The process drives at high temperatures the plasma arc produces, typically exceeding 2000°C. Plasma pyrolysis requires electricity as the primary energy source to create and sustain the plasma, distinct from thermal and thermo-catalytic methods. It also allows for precise control over the reaction conditions, leading to efficient conversion and higher purity of the products. Plasma pyrolysis stands out as the most expensive option among the three technologies. However, its reliance solely on electricity as an energy source, rather than using natural gas for heating like thermal and thermo-catalytic processes, allows for mitigating process emissions using renewable power sources. Despite its higher initial costs, this distinctive feature positions plasma pyrolysis as a more environmentally sustainable choice.
Each method has advantages and limitations, and the choice depends on cost, product quality requirements, and environmental considerations.
Key Features of NG Pyrolysis Vs SMR
The authors compare the existing steam methane reforming (SMR) process and the natural gas (NG) pyrolysis method for hydrogen production. In the SMR process, approximately 0.5 kg of hydrogen is produced per kg of methane, emitting 9–12 kg of CO2 equivalents per kg of hydrogen generated.
On the other hand, methane pyrolysis offers a low carbon footprint, with only 0.05 moles of CO2 produced per mole of hydrogen. Theoretically, one mole of methane yields one mole of carbon and two moles of hydrogen. Solid carbon production amounts to about 3 tons per ton of hydrogen from this perspective. To generate one tonne (1t) of hydrogen through methane pyrolysis, 4 tonnes of methane is required. Table 1 presents the comparison of NG pyrolysis with the SMR process.
Table 1: Comparison of SMR and NG Pyrolysis
Description | SMR | NG Pyrolysis |
Reaction Chemistry | CH4 + 2H2O —> CO2 + 4H2 | CH4 —-> 2H2 + C |
Moles H2 per mole CH4 | 4 | 2 |
kg of H2 per kg of CH4 | 0.5 | 0.25 |
Electricity Requirement (MWh/ton-H2) | 44.5 | 7.23 |
Process efficiency, (%) | 75 | 53 |
CO2 emission, kg-CO2/kg-H2 | 9 -12 | 2 – 3 |
TRL | 9 | 4-5 |
H2 Color Code | Grey | Turquoise |
Hydrogen derived from natural pyrolysis offers a significantly lower cost than green hydrogen, emitting 75% lower carbon emissions during production than SMR. Grey hydrogen currently carries an approximate price tag of 1.6–1.7 US$/kg-H2 when the natural gas price is 8 US$ per MMBtu. On the other hand, green hydrogen is projected to cost between 5 to 6 US$ per kg-H2. Among the different production methods, thermal pyrolysis is the most cost-effective, showing a levelized cost of hydrogen (LCOH) at 2.8 US$ per kg-H2. This cost assessment does not include any value attributed to the by-product. However, if the carbon by-product can be sold at 0.4 US$ per kg-solid carbon, the LCOH diminishes to 1.6 US$ per kg-H2 [4].
Key Recommendation to Scale-up
Several recommendations can be considered to scale up natural gas pyrolysis as a short-to-medium-term solution for hydrogen production.
- Investment in Research and Development: Allocate research and development resources to enhance natural pyrolysis technology’s efficiency and scalability. This includes optimising reactor design, improving catalysts, and increasing process automation to reduce costs and increase production yields. The temperature for pyrolysis varies depending on the catalyst utilised. For instance, Ni-based catalysts typically require temperatures ranging from 500 to 700 oC, while Fe-based catalysts necessitate temperatures between 700 and 900 o Carbon-based catalysts operate effectively within the temperature range of 800 to 1000 oC, whereas pyrolysis conducted without any catalyst typically occurs at temperatures ranging from 1000 to 1600 oC.
- Pilot Projects and Demonstrations: Perform a pilot study to evaluate the viability of hydrogen production through natural gas pyrolysis in an area with access to natural gas or methane gas and potential markets for hydrogen and carbon. Exploring non-catalytic thermal, catalytic-thermal, and plasma pyrolysis technologies for hydrogen production, focusing on the potential for catalytic-thermal pyrolysis to yield higher-value carbon by-products.
- Regulatory Support and Incentives: Support for regulatory frameworks and incentives to encourage investment in natural pyrolysis technology. This may include carbon pricing mechanisms, renewable energy mandates, or tax credits for hydrogen production from renewable sources.
- Infrastructure Development: Invest in developing the infrastructure necessary to support the production, storage, and distribution of hydrogen produced through natural pyrolysis. This includes building hydrogen refuelling stations, upgrading existing pipelines, and establishing supply chains for hydrogen transportation.
- Knowledge Sharing and Capacity Building: Facilitate knowledge-sharing and capacity-building initiatives to educate stakeholders about the benefits and potential of natural pyrolysis technology. This includes organising workshops, seminars, and training programs for industry professionals, policymakers, and the public.
- International Collaboration: Collaborate with international partners to leverage best practices, share technical expertise, and coordinate efforts to scale up natural pyrolysis technology globally. This can help accelerate innovation, reduce costs, and expand market opportunities for hydrogen produced through natural pyrolysis.
- Takeaways
Pyrolysis, a thermochemical process, holds significant promise for hydrogen production, mainly through the thermal decomposition of methane found in natural gas. This method offers simplicity, potential for high-purity hydrogen production, low carbon emissions, and scalability, leveraging natural gas’s abundance and accessibility. The primary aim of this article is to evaluate the feasibility of natural gas pyrolysis for hydrogen production compared to alternative technologies, considering energy requirements, CO2 emissions, costs, and other pertinent metrics.
Furthermore, exploring methane pyrolysis technologies (such as non-catalytic thermal, thermal-catalytic, and plasma pyrolysis) provides avenues for generating hydrogen, with catalytic thermal pyrolysis potentially yielding higher-value carbon by-products. Each technology presents unique advantages and challenges, underscoring the importance of pilot studies to determine the most suitable approach for India’s context. Scaling up natural pyrolysis requires investment in research and development, regulatory support, infrastructure development, knowledge sharing, and international collaboration to realise its potential as a short-to-medium-term solution for sustainable hydrogen production.
References
- Anders Holmen, Ola Olsvik, O.A. Rokstad, 1995. Pyrolysis of natural gas: chemistry and process concepts. Fuel Processing Technology, 42 (2–3), 249-267.
- Mark McConnachie, Muxina Konarova, Simon Smart. 2023. Literature review of the catalytic pyrolysis of methane for hydrogen and carbon production, International Journal of Hydrogen Energy, 48 (66), 25660-25682.
- Nuria S.B, Robert Schlögl, Holger Ruland. 2020. Methane Pyrolysis for CO2-Free H2 Production: A Green Process to Overcome Renewable Energies Unsteadiness. Chemie Ingenieur Technik 92 (10).
- Shashank R.P., et. 2023. A review of methane pyrolysis technologies for hydrogen production. Renewable and Sustainable Energy Reviews 181 – 113323.